Promethium, symbolized by Pm and assigned the atomic number 61, is a fascinating and rare element that occupies a unique position in the periodic table among the lanthanides. Named after Prometheus, the titan from Greek mythology who stole fire from the gods, Promethium is as elusive as it is intriguing. Its rarity stems from the fact that it is not found in significant quantities in the Earth’s crust and must be extracted from uranium ore or produced synthetically in nuclear reactors.
In the periodic table, Promethium is part of the f-block elements, known for their complex electron configurations and unique chemical behaviors. This article aims to explore the multifaceted aspects of Promethium, including its discovery and historical significance, distinctive physical and chemical properties, varied applications in modern technology, and the challenges associated with its handling and use. By delving into these topics, we hope to shed light on the importance and potential of this lesser-known element.
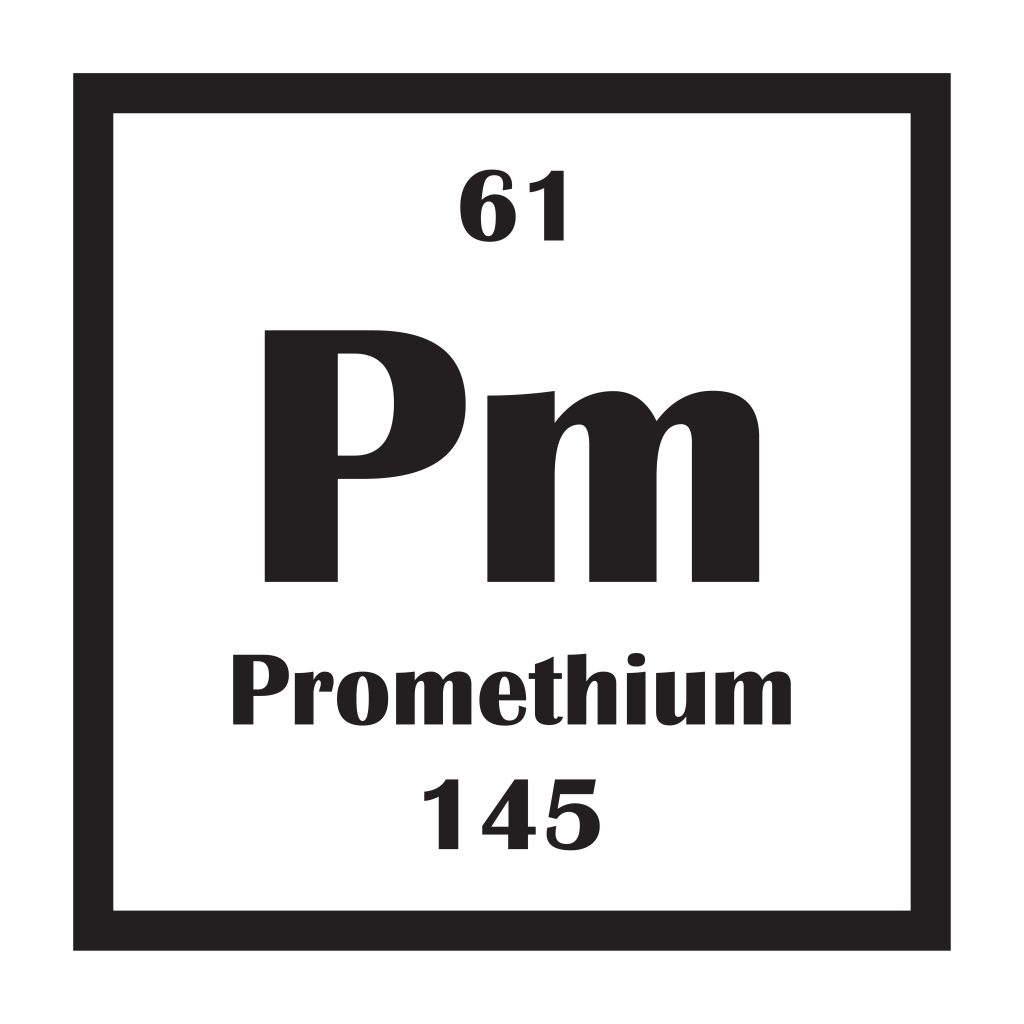
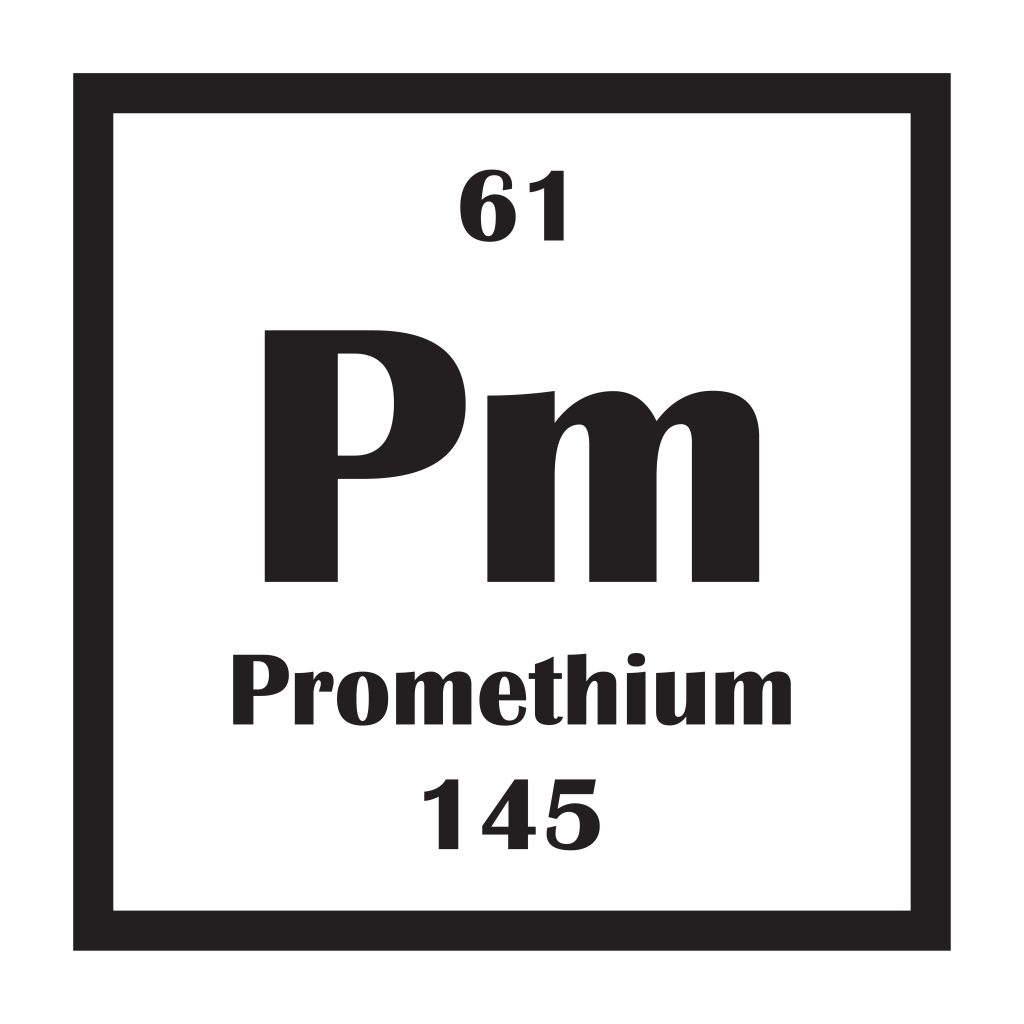
History and Discovery
The discovery of Promethium dates back to the mid-20th century, marking a significant milestone in the field of nuclear chemistry. Promethium was first identified in 1945 by a team of American chemists: Jacob A. Marinsky, Lawrence E. Glendenin, and Charles D. Coryell. Their work was conducted at the Oak Ridge National Laboratory, which was heavily involved in research during the Manhattan Project.
The discovery process began with the analysis of uranium fission products. As scientists studied the byproducts of uranium fission in nuclear reactors, they observed unexpected radioactive isotopes. Marinsky, Glendenin, and Coryell employed ion-exchange chromatography to separate and identify these isotopes. Through this meticulous analysis, they detected the presence of an element that had not been previously documented.
Initially, the newly discovered element was referred to as “element 61.” After confirming its existence and distinguishing its unique properties, the scientists decided to name it Promethium, inspired by the mythological figure Prometheus. This choice reflected the element’s fiery origin from nuclear reactions and its potential to unlock new scientific and technological advancements.
The identification of Promethium filled a missing spot in the periodic table and opened up new avenues for research in both fundamental science and practical applications. The discovery highlighted the importance of nuclear chemistry in expanding our understanding of the elements and their interactions, setting the stage for future innovations in the field.
Properties of Promethium
Promethium holds a unique position in the Lanthanide series, nestled between Neodymium (Nd) and Samarium (Sm) in the periodic table. Like other lanthanides, it is part of the f-block elements, which are known for their complex electron configurations and similar chemical properties. Despite these similarities, Promethium exhibits distinct characteristics that set it apart from its neighbors.
Physically, Promethium is a silvery-white metal that glows faintly in the dark due to its intense radioactivity. It has a melting point of approximately 1,042°C and a boiling point around 3,000°C, indicating a high degree of thermal stability. The atomic structure of Promethium is characterized by its electron configuration, [Xe] 4f^5 6s^2, which influences its chemical reactivity and bonding behavior.
Chemically, Promethium behaves similarly to other lanthanides, forming trivalent ions (Pm^3+) in most of its compounds. These ions typically exhibit similar reactivity to those of neodymium and samarium, forming various oxides, halides, and other compounds. However, due to its rarity and radioactivity, Promethium’s chemistry is less explored compared to more abundant lanthanides.
Promethium exists in nature only in trace amounts due to its instability, with all its isotopes being radioactive. The most notable isotopes are Promethium-145 and Promethium-147. Promethium-145 has a half-life of approximately 17.7 years and decays by electron capture to Neodymium-145. Promethium-147, the most stable and commonly used isotope, has a half-life of about 2.62 years and decays via beta emission to Samarium-147.
These isotopes of Promethium are of particular interest due to their radioactive properties. Promethium-147, for instance, is used in various applications, such as in nuclear batteries and thickness gauges, where its beta radiation can be harnessed effectively. Understanding the properties and behavior of these isotopes is crucial for their safe and efficient use in technological applications.
The unique physical and chemical properties of Promethium, coupled with its position in the Lanthanide series, underscore its significance in both scientific research and practical applications, despite its relative obscurity and challenges associated with its handling.
Occurrence and Extraction
Promethium is an exceedingly rare element, a characteristic that significantly impacts both its natural occurrence and methods of extraction. In nature, Promethium is found only in minute quantities, primarily as a byproduct of the spontaneous fission of uranium-238 and the alpha decay of europium-151. These processes yield trace amounts of Promethium isotopes, but not in concentrations high enough to warrant commercial extraction.
The scarcity of Promethium in the Earth’s crust can be attributed to its instability. All isotopes of Promethium are radioactive, with relatively short half-lives compared to the age of the Earth. This means that any primordial Promethium that might have existed during the planet’s formation has long since decayed into other elements. As a result, Promethium is not found in significant natural deposits like many other elements.
Given its rarity in nature, Promethium is primarily obtained through artificial means. The most common method of obtaining Promethium is as a byproduct of uranium fission in nuclear reactors. When uranium-235 or plutonium-239 undergo fission, a wide array of fission products are generated, including isotopes of Promethium. These isotopes can then be separated from other fission products using techniques such as ion-exchange chromatography and solvent extraction.
One of the main sources of Promethium extraction is spent nuclear fuel. After the fuel has been used in a reactor, it contains a mix of various fission products, including Promethium isotopes. The spent fuel is reprocessed, and Promethium is isolated from the mixture. This process not only provides a supply of Promethium but also helps in managing radioactive waste by extracting useful isotopes.
Additionally, Promethium can be synthesized by bombarding neodymium-146 with neutrons in a nuclear reactor, producing Promethium-147. This isotope is particularly valuable for its applications in nuclear batteries and other devices.
The extraction and purification of Promethium require sophisticated technology and stringent safety protocols due to its radioactivity. Despite these challenges, the controlled production of Promethium in nuclear reactors ensures a steady supply for research and industrial applications. Understanding the methods of Promethium extraction highlights the interplay between nuclear science and practical applications, illustrating the importance of advanced technologies in harnessing rare elements.
Applications of Promethium
Promethium, despite its rarity and radioactivity, has found several niche applications that take advantage of its unique properties. One of its primary uses is in the production of nuclear batteries, also known as radioisotope thermoelectric generators (RTGs). Promethium-147, the most stable isotope of Promethium, is utilized in these batteries due to its beta radiation. These batteries are highly valued in situations where long-lived, reliable power sources are needed, such as in space probes, satellites, and remote scientific instruments.
Another notable application of Promethium is in luminescent paints. When mixed with phosphors, Promethium-147’s beta particles can excite the phosphors to emit light, creating a luminescent effect. This property was historically used in glow-in-the-dark paints for watches, aircraft instruments, and other devices where visibility in low light conditions was crucial. However, the use of Promethium in such applications has decreased due to health and safety concerns, with safer alternatives being preferred.
Promethium also has potential uses in scientific research and advanced technology. Its radioactive properties make it useful as a beta radiation source in various types of equipment and experiments. For instance, it is used in thickness gauges for measuring material thickness in industrial processes. Its beta particles are also employed in certain types of X-ray machines and beta particle detectors.
Despite these valuable applications, there are significant limitations and challenges associated with the use of Promethium. The primary challenge is its radioactivity, which necessitates stringent handling and safety protocols to protect users and the environment from radiation exposure. Additionally, the extraction and production of Promethium are complex and costly, limiting its availability and widespread use.
Another limitation is the relatively short half-life of its isotopes. Promethium-147, with a half-life of about 2.62 years, requires regular replacement in long-term applications, adding to maintenance costs and logistical challenges. Furthermore, the disposal of Promethium-containing products must be managed carefully to prevent environmental contamination.
In conclusion, while Promethium’s unique properties enable it to serve specific high-value applications, its radioactivity, limited availability, and associated safety challenges restrict its broader use. Ongoing research and technological advancements may help to mitigate some of these challenges, potentially expanding the role of Promethium in scientific and industrial applications.
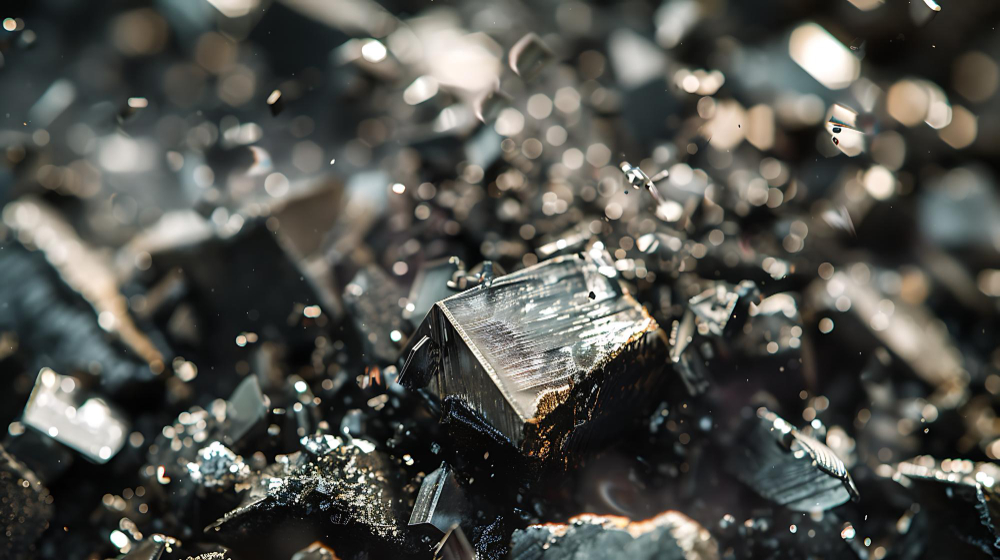
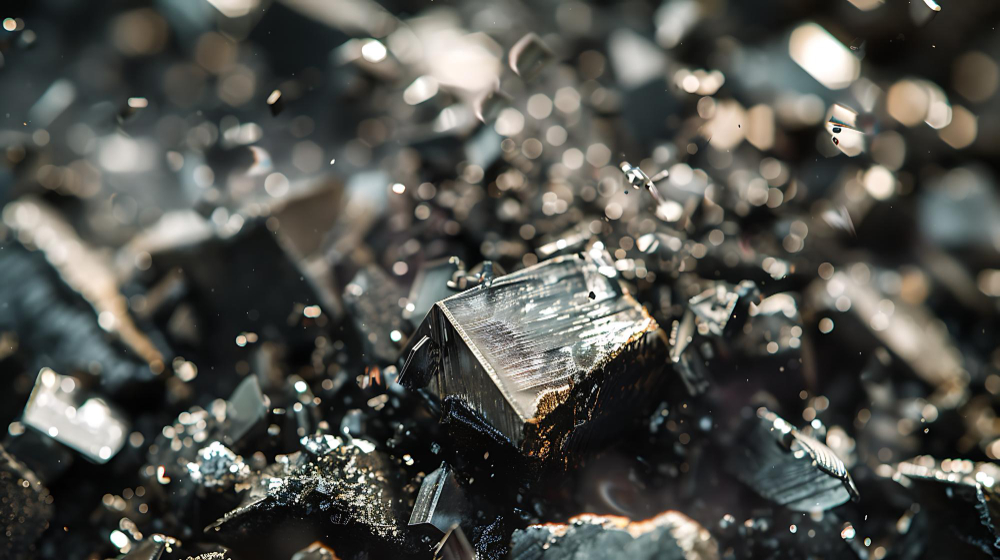
Health and Safety Considerations
The radioactive nature of Promethium necessitates rigorous health and safety protocols to protect individuals and the environment from potential harm. All isotopes of Promethium emit beta radiation, which can be hazardous if not properly managed. Beta radiation can penetrate the skin, causing radiation burns, and if inhaled or ingested, it can lead to internal radiation exposure, increasing the risk of cancer and other serious health issues.
Handling Promethium requires strict adherence to safety precautions to minimize exposure. Laboratory and industrial environments must be equipped with appropriate shielding materials, such as plexiglass or acrylic, to block beta particles. Workers must use personal protective equipment (PPE), including gloves, lab coats, and safety goggles, to prevent direct contact with the element. In addition, the use of fume hoods and proper ventilation systems is essential to prevent the inhalation of radioactive particles.
Storage of Promethium must also adhere to stringent safety standards. It should be kept in sealed, labeled containers that are stored in designated, secure areas. These areas must be monitored for radiation levels to ensure they remain within safe limits. Regular safety training and drills for personnel handling Promethium are crucial to maintaining a high level of preparedness and response capability in case of accidental exposure.
Potential health risks associated with Promethium exposure include radiation sickness, characterized by symptoms such as nausea, fatigue, and skin burns. Long-term exposure can increase the risk of developing cancers, particularly bone cancer, due to the element’s tendency to accumulate in bone tissue. To mitigate these risks, it is vital to implement comprehensive safety protocols, including regular health monitoring of workers, strict access controls to areas where Promethium is used or stored, and immediate medical response plans for any incidents of exposure.
By following these stringent health and safety measures, the risks associated with Promethium can be effectively managed, allowing its beneficial applications in science and technology to be realized while ensuring the well-being of those who work with this radioactive element.
Environmental Impact
The extraction and use of Promethium carry significant environmental implications that necessitate careful management and regulation. Due to its radioactive nature, Promethium poses potential risks to both ecosystems and human health if not handled properly. The primary environmental concerns revolve around the mining, processing, and disposal of materials containing Promethium, as well as potential contamination from its use in various applications.
Mining and extraction of Promethium typically occur as part of the processing of uranium ore or from spent nuclear fuel. These processes can generate radioactive waste, which must be meticulously managed to prevent environmental contamination. Radioactive waste disposal requires secure containment to avoid the release of harmful isotopes into the environment. Improper handling or accidental spills can lead to soil and water contamination, posing long-term ecological and health hazards.
To mitigate these risks, stringent regulations and guidelines have been established by national and international bodies. These regulations govern every aspect of Promethium’s lifecycle, from extraction and processing to transportation, storage, and disposal. For instance, the International Atomic Energy Agency (IAEA) provides comprehensive safety standards for managing radioactive materials, including Promethium. These standards aim to protect both people and the environment from the adverse effects of radiation.
In the United States, the Nuclear Regulatory Commission (NRC) oversees the safe use and disposal of radioactive substances, enforcing regulations that ensure facilities handling Promethium comply with rigorous safety protocols. Similarly, the European Union has implemented directives to manage radioactive waste and protect the environment from potential contamination.
Moreover, advances in technology and best practices in waste management are continually improving the environmental footprint of Promethium use. Recycling and reprocessing spent nuclear fuel, for example, not only recover valuable isotopes like Promethium but also reduce the volume of high-level radioactive waste requiring disposal.
Efforts to minimize the environmental impact of Promethium also include continuous monitoring and research to understand its behavior in the environment better. Environmental impact assessments (EIAs) are conducted for projects involving radioactive materials to evaluate potential risks and develop mitigation strategies.
In conclusion, while the extraction and use of Promethium present environmental challenges, the implementation of robust regulations and the adoption of best practices help manage these risks effectively. Through careful oversight and innovative approaches, the environmental impact of Promethium can be minimized, ensuring its applications benefit society while safeguarding our planet.
Summary
In summary, Promethium, with its symbol Pm and atomic number 61, stands out as a rare and fascinating element in the lanthanide series. Its discovery in the mid-20th century by Marinsky, Glendenin, and Coryell marked a significant advancement in nuclear chemistry, highlighting the complex interplay of scientific exploration and practical application. Promethium’s physical and chemical properties, including its notable isotopes such as Promethium-147, have enabled its use in specialized applications like nuclear batteries and luminescent paints, despite the challenges posed by its radioactivity.
The rarity of Promethium in nature necessitates its extraction from nuclear reactors, a process that underscores the intricate connection between nuclear technology and elemental chemistry. While Promethium’s applications in scientific research and industry are valuable, they come with stringent health and safety considerations due to its radioactive nature. Proper handling, storage, and disposal protocols are critical to mitigating potential health risks and environmental impacts.
The significance of Promethium in science and industry is profound, providing reliable power sources for remote and space applications and offering unique properties for specialized technologies. Looking ahead, ongoing research continues to explore new uses and safer handling methods for Promethium, potentially expanding its role in various fields. Advances in nuclear chemistry and waste management techniques promise to enhance the efficiency and safety of Promethium applications, paving the way for future innovations.
As we continue to uncover the potential of Promethium, it remains a testament to the ingenuity of scientific discovery and the importance of rigorous safety and environmental stewardship in harnessing the benefits of this remarkable element.